Lipid Oxidation- Initiation
Lipid oxidation is a sequential series of free-radical reactions involving unsaturated fatty acids and oxygen, leading to lipid degradation products and rancidity. During oxidation, free radicals act as intermediates that propagate lipid oxidation, eventually decomposing into reactive end products, including aldehydes, ketones, carboxylic acids, and non-reactive short-chain alkanes and alkenes. The free radicals, small polar aldehydes, and ketones then react with other food molecules (e.g., proteins, carbohydrates, and vitamins), decreasing their bioavailability, functional properties, and consumer acceptance. Oxygen addition onto a fatty acid double bond is not thermodynamically spontaneous, as molecular oxygen cannot directly react with the unsaturated double bond. Induction of a reactive species (e.g., fatty acid radical (L•) or activated oxygen species singlet oxygen (3O2) and reactive oxygen species (ROS) (hydroxyl radical (•OH), or superoxide radical anion (O2•-)). Three different induction methods exist for the oxidation of lipids, 1) enzymatic, 2) singlet oxygen oxidation, which relief on ROS, and 3) autooxidation. Lipoxygenase is a metalloenzyme containing iron that catalyzes the addition of oxygen to an unsaturated lipid forming lipid peroxide radicles and lipid hydroperoxides. The grains’ bran layers and beans contain lipids and lipoxygenase, which go rancid when unprocessed. Singlet oxygen oxidation requires a photosensitizer, usually containing porphyrins and porphyrin-like compounds, such as the heme group in hemoglobin or the porphyrin ring in chlorophyll, and UV light to create ROS, which directly reacts with the unsaturated fatty acids, after which it follows the same process as for autooxidation.
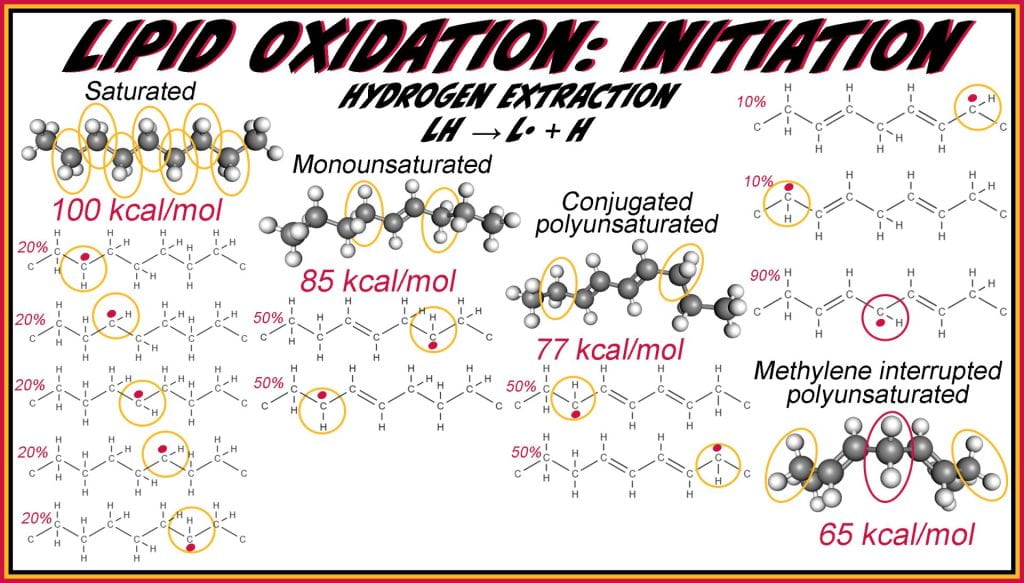
Autooxidation is the most prevalent mode of oxidation in food systems and occurs in three stages, initiation, propagation, and termination. Initiation forms the initial lipid radicals by chemical, enzymatic, or ROS. The rate-limiting step to oxidation is the initial hydrogen extraction. Hydrogens are most difficult to remove from saturated fats requiring ~100 kcal/mol, and the energy to dissociate the lipid decreases with increasing unsaturation.
Unsaturation allows for the delocalization of the pi bond, stabilizing the free radical long enough to form and further react. Two equally labile hydrogens exist on each monounsaturated fatty acid; oleic acid with a double bond at C9=C10 forms radicals at C8 or C11. Conjugated polyunsaturated fatty acids have double bonds spaced between single bonds, while methylene-interrupted fatty acids have two single bonds between unsaturation points. Conjugated unsaturated fatty acids, similar to monounsaturated fatty acids, have two equally labile hydrogens; again, both at one carbon removed from the double bond allow two initiation products to form. Conjugated polyunsaturated fatty acids (77kcal/mol) more easily form radicals because they delocalize across two unsaturated bonds compared to one double bond for monounsaturated fatty acids (85 kcal/mol). Finally, the most labile hydrogen is on methylene-interrupted fatty acids, and the hydrogen on the carbon between two double bonds is the major radical formed (65 kcal/mol), while the carbons adjacent to an outer double bond require more energy to extract and their radicals are a form less frequent.
Lipid Oxidation – Propagation
Once initiated, the free radicals spontaneously propagate in the presence of oxygen. In the case of methylene-interrupted fatty acid, linoleic (18:2 n9,12), the predominate radical is at C11 between the double bonds, while fewer radicals form at C8 or C14. At this point, the free radical (L•) is delocalized, and isomerization occurs where the radical occurs, maybe at C9, C11, or C13, allowing lipid peroxide radicals (LOO•) to form at each location upon spontaneous reaction with oxygen. Lipid peroxide radicals are short-lived and highly reactive, quickly scavenging hydrogen from other lipids, proteins, carbohydrates, DNA, and vitamins. The scavenging cascade propagates: 1) a lipid peroxide radical (LOO•) (removes hydrogen from lipid to become a lipid hydroperoxide ((LOOH)), 2) the lipid that lost hydrogen becomes a lipid radical (L•), 3) and reacts with oxygen to form another lipid peroxide radical (LOO•), 4) which in turn scavenges new hydrogen (LOOH) from another lipid peroxide repeating the cycle endlessly. As oxidation progresses, there is a continual loss in lipids’ unsaturation, coinciding with an increase in lipid peroxides, which are primary antioxidant products. Lipids hydroperoxides have lost the nutritional quality associated with lipid unsaturation; however, they are tasteless and odorless.

Lipid Oxidation – Termination
Several mechanisms exist as terminal, chain-breaking reactions, which remove the reactive products from the propagation reaction forming stable end-products. Lipid radical and lipid peroxide radicals can cross-link, forming covalent bonds between radicals (e.g., LOO• + LOO• orLOO• + L• orL• + L•.), resulting in secondary -oxidation products that are non-radicals (e.g., LOOOOL, LOOL, LL).

A highly unsaturated lipid can undergo reactions at each unsaturation point, forming multiple lipid peroxide radicals, which can transform into cyclized products. During termination, lipid hydroperoxides undergo beta-scission (chain-braking) reactions cleaving the unsaturated fatty acid between carbons adjacent to the peroxide radical into ternary oxidation products that include volatile (aldehydes, ketones, alcohols, acids, epoxides, short-chain alkanes and alkenes) and non-volatile (polymers of volatile small molecules ) compounds. The volatile products impart off-flavors and aromas, while the non-volatile polymers impart brown pigmentation. It is important to remember that minor radicals also initially form at C8 and C14 in addition to C11 and form radicals at C8, C9, C10, C11, C12, C13, and C14 due to radical isomerization. Complex mixtures of fatty acids allow for a broad, initial radical distribution generating a wide range of end-products, complicating the assessment of the extent of oil oxidation.
Factors Affecting Oxidation
Autooxidation and enzymatic oxidation kinetics depend on the amount and type of unsaturation, the presence of prooxidants (transition metals, hydrogen peroxide and ozone (O3)) and antioxidants, water activity, and the environmental conditions of temperature, UV light and oxygen exposure. An increase in the partial pressure of oxygen increases the dissolved concentration and the rate of lipid oxidation; thus, oxygen must be omitted during processing which is often displaced by nitrogen flushing the filled container before air-tight sealing.
Photochemical induction depends on electromagnetic characteristics, including light intensity and wavelength (UV is more active than visible), exposure length, temperature, and the presence of UV absorbers; oils are stored in dark glass bottles or opaque plastic vessels to counteract UV induction. Lipid autoxidation and LOOH decomposition are temperature dependent, whereby each 10 oC approximately doubles the oxidation rate; therefore, highly unsaturated oil should be refrigerated and not used in cooking applications. The chemical state of transition metal ions (e.g., iron and copper) alter their role in lipid oxidation. For example, oxidizing metals Fe3+ decompose LOOH significantly slower than reducing metals Fe2+ via the beta-scission of LOOH into free radicals via redox cycling pathways (Fenton Reaction). When oil is used as a heat-transfer media, high temperatures with oxygen exposure limit polyunsaturated oils when frying; however, long-term use of frying oils leads to lipid hydrolysis and fatty acid cleavage of even saturated fatty acids. Producing fatty acids and other oxidative and thermolytic products lowers the oil’s smoke point and autoignition temperature. Water absorbents are often added to frying oil to bind water released from the fried food to prevent fatty acid hydrolysis.

Antioxidants
Radicals are undesirable in foods and toxic to living organisms; in both cases, antioxidants slow radical formation or eliminate radicals after induction. Primary 1o antioxidants donate hydrogen to a lipid radical, terminating its propagation, and the radical antioxidant then forms a stable compound breaking the cascade.Secondary 2o antioxidants inhibit the formation of the initial free radical, slowing radical initiation. Both 1o and 2o antioxidants are used endogenously and in food systems.
In food systems, 1o antioxidants donate hydrogen from a hydroxyl, sulfhydryl, or nitryl group to the radical, forming a lipid or hydroperoxide. Oxidation of foods more readily occurs in the lipid phase, making water-soluble antioxidants less effective. To form a stable product, two 1o antioxidant radicals form a covalent bond, or the radical delocalizes across conjugated unsaturation, allowing for resonance stabilization of the antioxidant radical. Radicals are further stabilized by molecular features that provide large bulky electron clouds to shield the radical. Food systems contain natural (vitamins and phytochemicals) and synthetic (butylated hydroxytoluene (BHT), a synthetic, highly effective antioxidant) compounds. 1o antioxidants include fat- (Vit A, Vit E, Vit K) and water (Vit C) soluble vitamins and phytochemicals (organosulfur compounds high in garlic and cruciferous vegetables, carotenoids in tomatoes and carrots, and polyphenols in apples). Endogenous cellular enzymes include glutathione peroxidase (GHS-P) and glucose 6-phosphate dehydrogenase shuttle electrons, often using minerals such as zinc and selenium to shuttle electrons. Other 1o antioxidants that have endogenous roles include uric acid, glutathione, and Co-enzyme Q10 at sequencing free radicals.
Secondary 2o oxidants have more variability in structure and inhibition mechanisms. Cellular enzyme superoxide dismutase (SOD) and catalase (CAT) are singlet oxygen quenchers that convert reactive oxygen species (ROS) to water and molecular oxygen, while glucose oxidase scavenges oxygen during glycolysis while breaking down glucose to ATP, water and CO2. Oxygen scavengers consume oxygen, preventing the formation of ROS, while singlet oxygen quenchers transform ROS back to oxygen and water. Iron can behave as a prooxidant (e.g., heme groups) or an oxygen-scavenging antioxidant depending on its chemical state through the Fenton reaction, while chelators remove prooxidant transition metals. UV absorbing molecules are highly conjugated, prevent UV from initiating oxidation and include various plant pigments (e.g., chlorophyll and lycopene).

Endogenous Biological Antioxidant Mechanisms
Exogenous (foods you eat) and endogenous (biological and cellular) mechanisms are essential to prevent oxidative damage as radicals can react with proteins such as enzymes, carbohydrates anchored to cell walls, lipids in bilayers, and DNA leading to carcinogenesis and mutagenesis. Radicals and prooxidant reactive oxygen species (ROS) become toxic to cells when not sequestered. To prevent ROS from initiating oxidation, superoxide dismutase (SOD), an enzyme found in the cellular cytoplasm of cells, peroxisomes, and mitochondria, converts superoxide to hydrogen peroxide, which is hydrolyzed into water and oxygen by catalase (CAT) or glutathione peroxidase (GSH-P). Glutathione (GHS) is biologically essential and found in most cells to protect against oxidative damage by reducing lipid hydroperoxides to water and lipid alcohol (LOH) or by donating electrons to lipid radicals, again forming LOH. GHS (L-cysteine, L-glutamic acid, and glycine) donates electrons by forming GHS dimers (GSSG) via a disulfide bridge between cysteines. It is very important in the body and is crucial in protecting cells from oxidative damage. GHS is returned to its original state from GSSG after receiving a pair of electrons as NADPH converts NADP+, breaking the disulfide bond. NADPH is replenished via the HMP shunt and glycolysis, which is the metabolism of glucose in the presence of oxygen (oxidative phosphorylation) to produce ATP. Exogenous antioxidants, often added during food formulation to slow the initiation and propagation of radicals, are essential for preserving the nutritional and sensory qualities of foods and ingredients high in polyunsaturated oils. The role of dietary antioxidants in the sequestering radicals within the body is less well understood and characterized.
