Structure of Carbohydrates

Carbohydrates range from small to very large molecules comprised of carbon, hydrogen, and oxygen atoms and are ubiquitously found in whole and processed foods, which are either digested and metabolized to glucose which the body can immediately utilize as an energy source, or be stored for later use, or fermented by the gut microflora. The specific structure of carbohydrates is predetermined during biosynthesis, which can include post-translation modifications. Small mono-and disaccharides have colligative properties, increasing the boiling point and decreasing the freezing point of water, and reducing water activity or fugacity. Oligo- and polysaccharides do not have colligative effects, but they are highly functional, holding cell walls together, as ingredients modifying viscosity (alginates and pectins), forming glassy foods such as instant pasta (amylose and amylopectin), and creating gels (pimento added to pitted olives).
Polysaccharide Structures

Polysaccharides (>10 monosaccharides) are an incredibly diverse group of macromolecules ubiquitously found in all living systems and essential for cell wall adhesion, cell signaling, and energy storage. Food polysaccharides are techno-functional ingredients that alter the viscosity and form gels and are typically obtained from plants, animals, algae, fungi and bacteria. Polysaccharides are subdivided if they are homopolysaccharides, containing a single monosaccharide unit (starch, glycogen, inulin, agar, cellulose), or heteropolysaccharides, with different sugars and covalent linkages (pectin, alginate, agaropectin), and if they are linear (amylose, inulin) or branched (amylopectin, glycogen, agaropectin, xanthan). They are also differentiated based on their digestibility as either glycemic (starch, glycogen) or non-glycemic (alginate, agar, pectin), broadly classified as soluble and insoluble fibers.
Polysaccharides and Viscosity
How polymers and, ultimately, polysaccharides modify structure is not based on collective effects; instead, they increase viscosity, stabilize emulsion and foams, and solidify water into gels due to their large molecular weight and ability to interact with other polysaccharide molecules. The number of monosaccharide subunits covalently bound into a polysaccharide is proportional to the increase in viscosity; longer polysaccharides increase viscosity more than oligosaccharides and small polysaccharides. In solution, polysaccharides are flexible and often adapt a supramolecular arrangement shorter than the molecular length, whereby the smallest enclosing sphere is the hydrodynamic radius (HR), where only water and low molecular mass solutes can reside alongside the polymer chain.
First, consider a linear polysaccharide; in solution, the long, flexible chains adopt an arrangement allowing folding and are enclosed within a hydrodynamic radius, where molecules larger than water are excluded. The larger the hydrodynamic radius of a polysaccharide, the greater the increase in viscosity and ability to form a glass. Two polysaccharides of equal molecular mass that only differ by the presence of branching (amylose (linear) compared to amylopectin (branched)) have altered hydrodynamic radii, where the branched polysaccharide occupies a smaller hydrodynamic radius. Due to the difference in HR, linear polysaccharides tend to have lower molecular density but a greater effect on enhancing viscosity. A branched polysaccharide with the same molecular mass has a lower hydrodynamic radius, which is inversely proportional to the branching rate, and impedes viscosity enhancement or gel formation.

The other equally important consideration is how the polysaccharide chains interact at low concentrations. Polysaccharides that form covalent junctions between each other have the greatest increase in viscosity and are non-reversible. Most food polysaccharides rely on non-covalent interactions (e.g., ionic > hydrogen > van der Waals) combined with entanglement, which forms a bicontinuous water and polymer network, which may or may not be reversible. To summarize, molecular weight, concentration, the addition of an inert filler, or increased pressure each increase the viscosity of polysaccharide solutions. Conversely, viscosity decreases with increased branching, hydrolysis, temperature, plasticizer (lower molecular weight molecules), or lubricant (in foods, often fats and oils)).
Common Homopolysaccharides

Homopolysaccharides have a repeating monosaccharide unit covalently bound by glycosidic bonds; linear homopolysaccharides have a single orientation glycosidic bond, while branched homopolysaccharides have two or more orientations of glycosidic bonds. The primary animal storage polysaccharide for glucose is glycogen bound in linear chains via alpha (1 to 4) and branch point via alpha (1 to 6) glycosidic bonds. In plants, glucose is stored in starch granules containing amylose and amylopectin; amylose is linear with only alpha (1 to 4) glycosidic bonds, and amylopectin is branched with alpha (1 to 4 & 1 to 6) bonds. The only difference between amylopectin and glycogen is the frequency of occurrence of branching. Glycogen, amylose and amylopectin are all glycemic carbohydrates, as digestive enzymes hydrolyze their glycosidic bonds and glucose is obtained.
Non-glycemic homopolysaccharides contain Beta-conformers such as cellulose (monomers: glucose, glycosidic bond: beta (1 to 4)), inulin (monomer: fructose, glycosidic bond: Beta (2 to 1)), and beta-glucan (monomer: glucose, glycosidic bond: beta (1 to 3 & 1 to 6). Beta-glucan, found in cereals such as barley, oats, wheat, and inulin, in onions, bananas, and garlic, are considered bioactive, meeting the Food And Drug Act soluble fiber requirements. For example, if oat flour or oatmeal has > 4 % (dry weight basis (dwb) moisture) of beta-glucan and > 10 % dwb total dietary fiber, the food can be labeled as a “good source of fiber.” Health Canada requires an energy gain of 2 kcal/g for all nonglycemic but fermentable carbohydrates, including inulin, in calculating the calories per serving. Inulin is also commonly hydrolyzed to produce fructooligosaccharides, adding health benefits to the gut microbiome and being an alternative sweetener.
Structure and Gelation of the Homopolysacchairde: Starch
During photosynthesis, excess glucose cannot be stored in its monomeric form due to its colligative nature, and to overcome this, plants polymerize glucose into starch polymers. Glucose synthesized in the chloroplasts is then transported and polymerized in amyloplasts, stored as starch granules. Starch is a homopolymer containing only glucose, which may be linear (amylose) or branched (amylopectin); the glycosidic bonds along the linear segments are alpha (1 to 4) and branch points introduced via alpha (1 to 6) glycosidic bonds. Depending on the plant, starch is generally distributed from 20 to 25% amylose and 75 to 80% amylopectin by dry weight; however, GMO corn can get as high as 70% amylose. Six of the world’s staple crops have unique amylose: amylopectin ratios depending on the cultivar, which translates to unique physical properties. While corn, potato and wheat starch dominate Western societies, much of the world relies on rice, plantains (banana) and sorghum starches. Starches derived from each staple commodity have differing starch granules characteristics (e.g., amylose to amylopectin ratio, amorphous to crystalline band ratio, size (chloroplasts have sub-micron elongated granules while potatoes contain huge oval shaped granules), shape, and supramolecular granule architecture). Two carbohydrate fraction ingredients are obtained depending on how the raw agricultural commodity is processed. Flour is ground and may be refined or whole and contains mostly starch but also has protein and lipids. Starch is further processed to eliminate the protein, lipid, and other non-starch fractions purifying only starch granules. Starch is a more efficient thickener than flour due to the lipids and protein absence, altering physical properties and opacity.
Starch granules, the plant organelles where starch is produced and stored, comprise layered amorphous and crystalline regions of densely packed amylopectin and amylose molecules strongly interacting via hydrogen bonding. In cereals, starch granules are found in the seed endosperm, while in tubers, such as potatoes, granules are concentrated in parenchyma cells. The long linear glucose alpha (1 to 4) segments between branch points of amylopectin adopt a helical conformation that rotates around a central axis. Amylopectin, depicted beside the large starch granule, form dense regions of helical coils that efficiently pack together into semicrystalline regions (dark bands depicted in granule); while the amorphous regions (light bands) contain amylopectin branch points that have less order. Starches, mainly acquired from tubers and cereals, vary in the ratio of amylose to amylopectin and in techno-functional properties, including how quickly and how much the starch thickens or increases the viscosity, the quality of the thickening, and their organoleptic properties, thus, to attain the desired physical properties requires selecting the appropriate starch by understanding the properties of how each starch behaves.
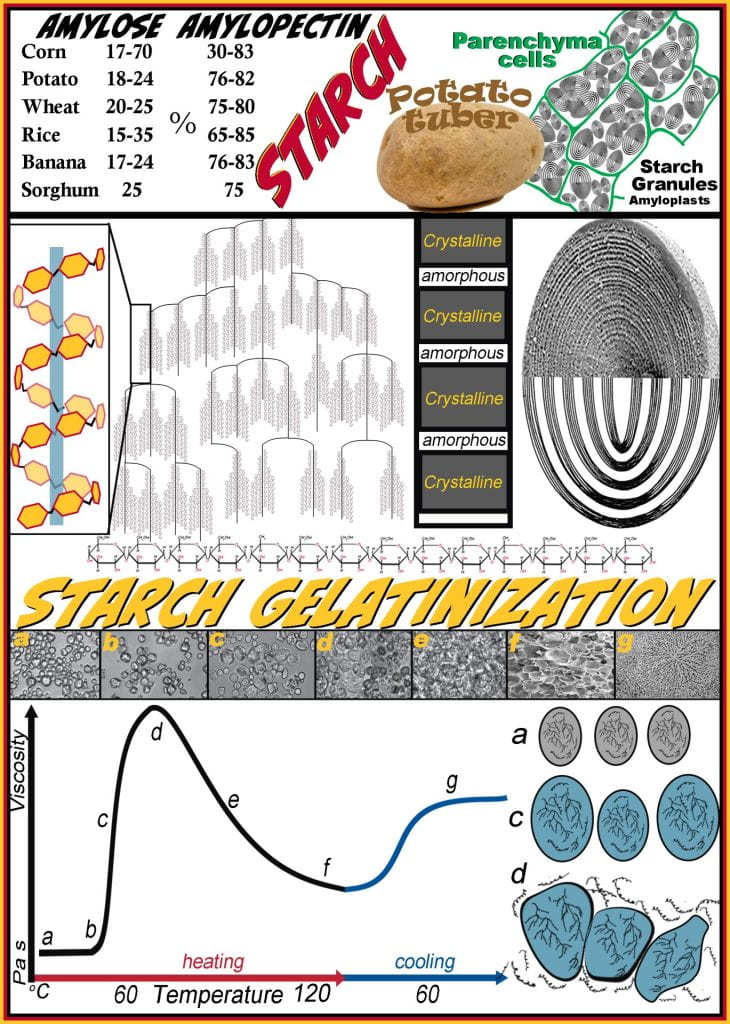
Corn starch added to water ((a) in image and figure) remains dense granules because water cannot imbibe the granule due to the strong intermolecular hydrogen bonding between sugar monomers in the polymer. Recall that hydrogen bonding strength is at its maximum near 4 oC and decreases as temperature rises. Until heat is applied, granules remain intact and suspended but do not interact strongly with water. Most starches begin to gelatinize (b) above 60oC; at this point, the hydrogen bonds weaken between polysaccharides, allowing water to exchange non-covalent interaction, causing the granule to swell as water is incorporated. Continued heating (c) above the gelatinization temperature causes more water to absorb into the granule, causing it to swell but remain intact. Viscosity is rapidly increasing (b-c-d) because the hydrodynamic radius of the granules is increasing simultaneously. The maximum viscosity is achieved when the starch granules have swelled, allowing their ends to come into contact with other granules while remaining intact; as this occurs, amylose leaches from the granules into the aqueous phase. At this point (d), a starch gel is achieved and very sensitive to continued heating (e) or over-mixing, as this causes granules to rupture, leaching all of the starch into the water, and the structure imparted by the contact between granules is lost causing the viscosity to decline rapidly until a starch paste is achieved. When making pudding or gravy, it is common to be over-mixed, causing them to be runny. Cooling a starch gel (from d) or paste (from f) causes an increase in the viscosity caused by a strengthening between amylose and amylopectin molecules. The strengthening of the network can be observed for pudding stored for long periods at refrigerated temperatures causing a thick film to form on the surface.
Structure and gelation of the Homopolysacchairde: Agar
Amylose is the simplest homopolysaccharide, with a single monomer and glycoside bond type. Agarose, used in plating bacteria and with many applications in food science, is a much more complex polysaccharide but still a homopolysaccharide with a repeating unit that is a disaccharide instead of a monosaccharide. Disaccharides are the smallest subunit of a polysaccharide.

Red seaweed, a form of algae, is specifically harvested for its polysaccharide fraction Agar Agar, a combination of agaropectin and agarose. Agraropectin does not gel and is separated from agarose, a polymer of the disaccharide agarose (D-galactose and galactopyranose bound via beta (1 to 3)). The polysaccharide agarose comprises agarose monomers with covalently bound alpha (1 to 4) glycosidic linkages resulting in a linear polysaccharide. Upon heating in solution, the chains increase flexibility and form interactions with water (e.g., become hydrated), forming a viscous solution, and upon cooling and strengthens hydrogen bonding, helical segments form between two or more polysaccharides resulting in a bicontinuous network of water and polysaccharide (weak gel) and over time or reheating the interactions strengthen and strong gel results.
Structure of Heteropolysacchairdes
There are countless heteropolysaccharides, most of which are soluble or insoluble fibers isolated from plants, algae, bacteria, fungi and animal by-products. Heteropolysaccharides contain more than one type of monosaccharide, glycosidic bond, often leading to branched chains; additionally, sugars can be modified, which can be covalently bound to an amino acid or lipid. Those with functional or bioactive properties are often isolated and purified as gums, mucilage, and other hydrocolloids. Bioactives chitin and chitosan are isolated from waste shells of shrimp and crabs and are promoted for wound healing and as an anticoagulant, anti-ulcer, and anti-microbial. While heparin, isolated from mast cells, is a potent anticoagulant. Functional ingredients, such as pectin, alginate and carrageenan, all heteropolysaccharides, have numerous ingredient applications used as thickeners and emulsion stabilizers which, upon consumption, are non-caloric and instead are fermented by the gut microbiome. Examples include the addition of carrageenan due to its stabilizing effect on fat droplets and protein aggregates in chocolate milk, the use of alginates to make the pimento for pitted olives, and pectins widely used to make Jams and Jellies.
Structure and Gelation of the Heteropolysacchairde: Pectin
Pectin is a complex polysaccharide found in the cell walls of plants and is commonly used to gel jams, jellies, and gummy candy. Following juicing, most commercially available pectin is valorized from fruit peel (citrus (oranges, lemons, limes)). During extraction, it is important to blanch the source to eliminate pectin pectinesterase (PE) or pectin methylesterase (PME), which hydrolyze the polymer resulting in a drastic decrease in viscosity. Pectin polysaccharides contain smooth (homogalacturonan) and hairy (rhamnogalacturonan) regions. The smooth region contains homogalacturonan, a linear polymer segment containing the oxidized sugar galacturonic acid linked via alpha (1 to 4) glycosidic bonds, where oxidation introduces carboxylic acid (COOH) groups on the sugar molecules. The second region is the hairy region with numerous branch points with side chains of galactose, arabinose, and other molecules bound via alpha (1 to 4) and alpha (1 to 2) glycosidic bonds. Pectin, therefore, contains alternating regions of homogalacturonan and rhamnogalacturonan and ranges in size from 50 to 150 kDa. Pectins can be classified as low and high methoxy pectins, where the hydrogen on the hydroxyl group of the carboxylic acid is replaced with a methyl group which requires different gel conditions. Pectin is “graded” according to the degree of methoxylation/esterification. Most pectin, when extracted, has a degree of esterification between 50-80%.

Low-methoxy pectin has less than 50 % of galacturonic acid –COOH groups methoxylated. Low methoxy pectin infers that the oxidized homogalacturonan contains carboxylic acids (COOH), which deprotonate at pH above 3.2. Once the carboxylic acids are deprotonated, the negative charge can form a salt bridge via divalent ions (Ca2+ or Mg2+) between two pectin polymers, preventing electrostatic repulsion, forming a strong jam without any other added ingredients and is used to make no-sugar-added jams and jellies. High-methoxy pectin has more than 50 % of galacturonic acid –COOH groups methoxylated and under acidic pH (2.8 < pH < 3.2), ensures that the carboxylic acid groups are protonated to guarantee there is no electrostatic repulsion between polymers. However, in the absence of salt bridges, high-methoxy pectin requires 65-70% sugar to bind water to prevent interference with pectin-pectin hydrogen bonding and gel formation.
Structure and Gelation of the Heteropolysacchairde: Alginate
Alginate, obtained from brown seaweed, is a naturally occurring anionic linear polymer used in food and biomedical applications due to its biocompatibility, low toxicity, and relatively low cost. Alginate is a linear, unbranched copolymer of (1→4)-linked β-D-mannuronic acid (M) and α-L-guluronic acid (G) in a 2:1 M: G ratio. The disaccharides occur in blocks along the polysaccharide where G blocks contain repeating α-L-guluronic acids, M blocks repeat β-D-mannuronic acids, and MG blocks alternate between disaccharides. Within the long G blocks, two guluronic acids, side by side-by-side, form a “cavity” with the carboxylic acid groups on the opposite sides; in the presence of Ca2+ alginates form strong ionic bonds with the deprotonated COO– groups on guluronic acid and gel almost instantaneously. Calcium interactions between G-blocks form a strong gel as multiple alginates form ‘egg boxes’ where calcium is located between ionized groups forming many salt brides between multiple polymers of alginate. Alginate regions containing M or MG blocks do not engage in salt bride formation and are contained in the flexible polymer regions between ‘egg boxes.’
