Structure of Carbohydrates
Carbohydrates range from small to very large molecules comprised of carbon, hydrogen, and oxygen atoms and are ubiquitously found in whole and processed foods, which are either digested and metabolized to glucose which the body can immediately utilize as an energy source, or be stored for later use, or fermented by the gut microflora. The specific structure of carbohydrates is predetermined during biosynthesis, which can include post-translation modifications. Small mono-and disaccharides have colligative properties, increasing the boiling point and decreasing the freezing point of water, and reducing water activity or fugacity. Oligo- and polysaccharides do not have colligative effects, but they are highly functional, holding cell walls together, as ingredients modifying viscosity (alginates and pectins), forming glassy foods such as instant pasta (amylose and amylopectin), and creating gels (pimento added to pitted olives).
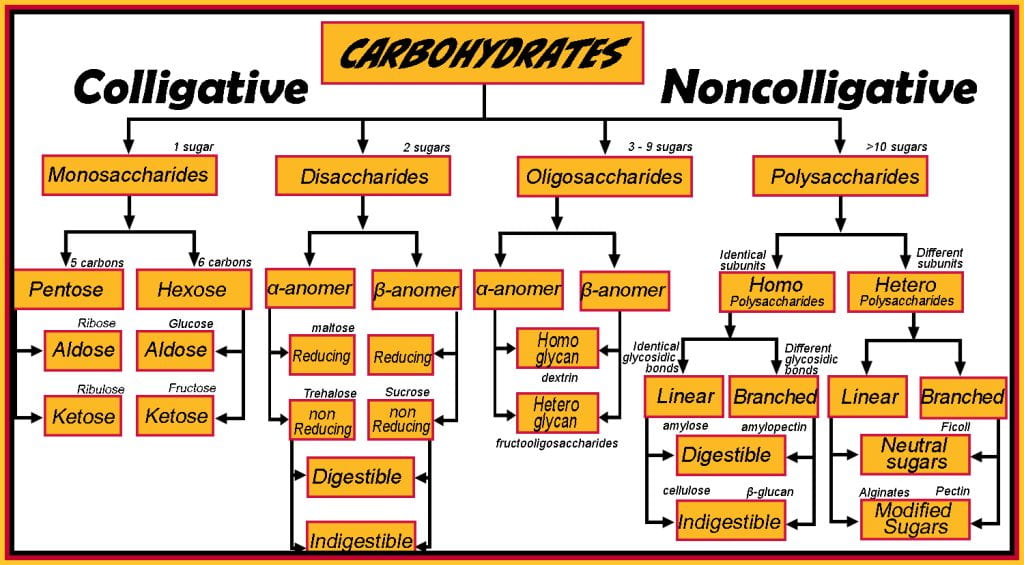
Structure of Monosaccharides
Monosaccharide carbon length ranges from three (triose) to seven (heptose) and is the smallest carbohydrate that comprises larger disaccharides (2 monosaccharides), oligosaccharides (3-10 monosaccharides) and polysaccharides (>10, kDa). While all length sugars are important, the focus herein is on hexose; others, such as pentoses, are beyond the scope of this course but are essential as ribose and deoxyribose are part of DNA. Hexose sugars have five alcohol groups, each on a different carbon, and the final carbon is a carbonyl. If the carbonyl is at the primary position, it is an aldehyde and termed an aldose sugar; if the carbonyl is at the secondary position, it is a ketone, and the sugar is a ketose.

The configuration of a hexose sugar has 4 chiral carbons, and their stereochemistry differentiates individual monosaccharides (glucose, galactose, mannose and fructose) and their enantiomeric (D or L) forms. Examples of different configuration stereogenic centers are first, D-glucose and L-glucose; these two molecules are mirror images of each other and are enantiomers; these are differentiated from diastereomers, which have a different arrangement of their centers, as would be the case of D-galactose compared to either D or L-glucose. The takeaway is that monosaccharide biosynthesis controls configuration: 1) carbon length, 2) carbonyl position, and 3) stereogenic placement of alcohols chiral carbons (e.g., enantiomers and diastereomers). Conformation is highly dependent on the environment (temperature, pH, solvent) and occurs when sugars form a cyclic ring between carbon sn-1 and sn-5, in the case of D-glucopyranose, termed alpha (a) and beta (b) anomers. Although this seems trivial, the enzyme secreted along the human digestive tract primarily hydrolyzes covalently linked alpha sugars; one exception is lactase. Another important aspect of anomers is their reducing ability which can be conferred to disaccharides through polysaccharides.
Structure of Disaccharides
Disaccharides comprise two monosaccharides (e.g., sucrose is glucose and fructose; lactose has glucose and galactose; and maltose is two glucose molecules) that covalently bond via a condensation reaction forming a glycosidic bond and differ based on the monosaccharides, the position of the functional groups involved in forming the glycosidic bond, and the anomeric forms. Disaccharides with (alpha)-glycosidic linkages between the a-anomeric carbon of one monosaccharide unit and the hydroxyl group on the fourth carbon of the other monosaccharide unit create a relatively straight (alpha(1->4)) linkage, which, when polymerized in polysaccharides allow the disaccharides units to pack (e.g., amylose and amylopectin) closely. Disaccharides with (beta)-glycosidic linkages form the glycosidic linkage between a b-anomeric carbon and the hydroxyl group on the first carbon of the second monosaccharide creates a relatively bent bond, making it more difficult to pack closely together. These slight differences in orientation alter how the body digests and metabolizes them.
Some disaccharides are hydrolyzed along the human gastrointestinal tract: sucrose, lactose, maltose, and trehalose. They are broken down by brush border enzymes (sucrase, lactase, maltase, trehalase) into their monosaccharides along the small intestine, which are absorbed and used for energy. At the same time, lactulose has the same beta(1 -> 4) bond as lactose, but humans lack a digestive enzyme for lactulose.

Fibers such as hemicellulose and cellulose are polymerized cellobiose; again, humans lack a digestive enzyme for and why they are non-caloric. Although humans lack a digestive enzyme to hydrolyze fibers, the gut microbiome contains many more enzymes that utilize non-digestible carbohydrates, often forming a symbiosis between host and guest microorganisms. Understanding the differences between disaccharides helps digestion and metabolism and should inform dietary choices.
Reducing Sugars
When sugars cyclize, they contain either an acetal (R2C(OR’)2) or a hemiacetal (R1R2C(OH)OR) group; the difference being there is a hydroxyl group one carbon from the glycosidic bond (oxygen in the central carbon ring) for reducing sugars; while for nonreducing sugars the is no hydroxyl group one carbon removed from the glycosidic bond. Reducing sugars reduce other compounds and, in the process, are oxidized. The hemiacetal group allows conversion between the linear and cyclic forms in alkaline pH, and in the linear form, aldehydes or ketones (ketose sugars undergo tautomerization before they contain an aldehyde and are considered aldoses sugars) act as reducing agents. Therefore, reducing sugars must contain a hemiacetal that allows the ring to open, adapting the open-chain form with an exposed aldehyde.

Reducing sugars include all monosaccharides and some disaccharides depending on the position of the glycosidic bond formed between the monosaccharides. Common dietary monosaccharides galactose, glucose and fructose, and disaccharides lactose and maltose are all reducing sugars. Nonreducing disaccharides (sucrose and trehalose) have both anomeric carbons engaged in the glycosidic bonds and do not convert to an open-chain form with an aldehyde group. Reducing disaccharides like lactose and maltose have only one of their two anomeric carbons involved in the glycosidic bond, while the other is free and can convert to an open-chain form with an aldehyde group. Oligosaccharides and polysaccharides contain a reducing end; however, other covalently bound sugars along the chain are nonreducing sugars; therefore, their reducing potentials are low and considered nonreducing. The delineation between reducing and nonreducing sugars is important because reducing sugars react with amino acids and partake in the Maillard reaction (toasting bread, coffee, and cocoa), while nonreducing sugars, such as sucrose, do not.
Maillard reaction is a sequence of reactions that occurs while cooking food high in protein and reducing sugars at high temperatures, which is important in determining the flavor and color of food. Also, the ability to measure the reduced sugar content is central to the manufacture of maltodextrin, corn and glucose syrups. As starches hydrolyze into smaller units, each unit has a reducing sugar end, so if an amylose starch molecule is split in two, it goes from having one reducing sugar to each fragment having its own; thus, two reducing sugars are formed, and so on until the desired dextrose equivalence (DE) is reached. Reducing sugars react with amino acids in the Maillard reaction.
Benedict’s Test for Reducing Sugars
Benedict’s reagent tests for reducing sugars using copper (II) sulfate, sodium carbonate, and sodium citrate; sodium citrate is a chelating agent that inhibits precipitation of cupric hydroxide; sodium carbonate ensures a basic pH allowing the ring to open, exposing the aldehyde group and when heated in the presence of reducing sugar, the copper (II) sulfate is reduced to copper (I) oxide, while the carbonyl is oxidized to a carboxylic acid and through the reaction the solution becomes brick-red, and the intensity is proportional to the amount of reducing sugar. Benedict’s test measures the presence of sugars in urine and in the food industry to test for the presence of sugars in products such as fruit juices and jams. Tollens’ test distinguishes reducing sugars with an aldehyde or a ketone; aldehydes are readily oxidized, whereas ketones must first tautomerize and do not partake in the reaction. Tollens’ reagent is a colorless, basic, aqueous solution containing silver ions coordinated to ammonia [Ag(NH3)2+] and the presence of a reducing sugar causes a reduction of silver ions in Tollens’ reagent, creating a metallic silver mirror on the surface of the glass test tube.