Protein Denaturation
Protein denaturation is important for many reasons and refers to the loss of 3D conformational order. Proteins are denatured when the secondary (2o) and tertiary (3o) structures are altered, but the primary structure is unaffected as peptide bonds between amino acids remain intact. Globular tertiary structures formed by proteins are often enzymes (insulin and pepsin) and more sensitive to denaturants, while fibrous proteins are more structural (e.g., collagen and silk).
Denaturation of Globular Proteins – Enzymes
Globular endogenous enzymes found in all organisms are essential for life and, after that, during decomposition. Enzymes are functional due to the spatial arrangement of amino acids, for example, amino acids Asp, His, and Ser in the catalytic triad of hydrolytic enzymes, where the function of the enzyme is reliant on the proper and exact folding of the protein to ensure that the substrate can access the catalytic site and preserves its function. Due to the importance of enzymes in cellular function, denaturing them results in cell death; proteins readily denature when applying heat (thermal processing), curing (chaotropic), fermenting (ethanol) and acidifying (lactic and acetic acids). Denaturaturants, techniques or molecules that cause denaturation of proteins can be broadly subdivided into six categories: 1) temperature; 2) pH (often to pI); 3) disulfide bond reduction; 4) solvent polarity (hydrophobic effect); 5) ionic charge alteration (chaotropic); and 6) cross-linking (chemical: glutaraldehyde; physical: kneading dough). These techniques alter the type, occurrence and strength of non-covalent interactions along peptide backbones and between their amino acid side (R) groups, thereby altering the overall electrostatic charge, causing the protein to fold differently. When the enzymes denatured are microbial, if they are present in food, they will no longer be viable and able to replicate.
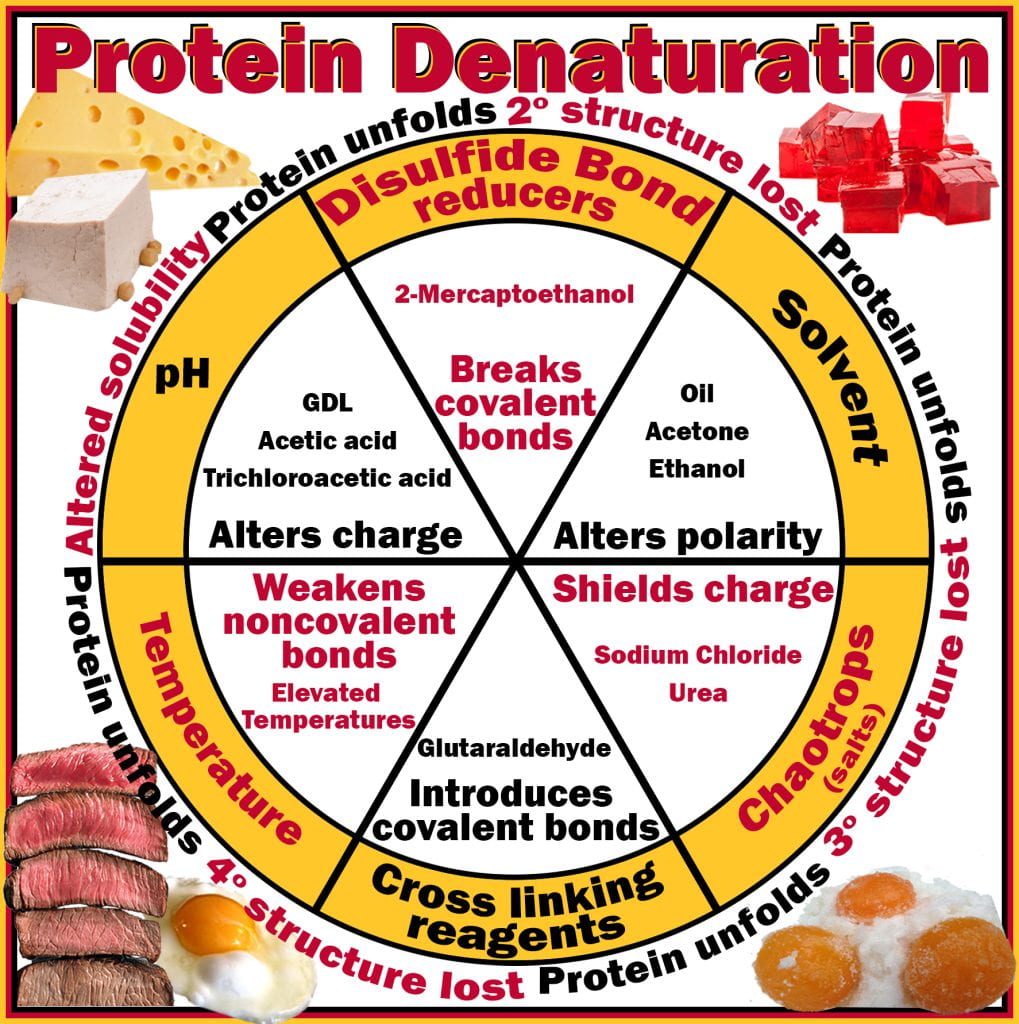
During food processing, thermal and non-thermal (uv light, high-pressure, electric current, irradiation, chemical) processes degrade and inactivate enzymes eliminating pathogenic and spoilage bacteria. Endogenous enzymes found in animal and plant food products are often deteriorative, and denaturing them prevents the reaction and deterioration of food quality. Blanching is a thermal process for many plant products before packaging to eliminate the activity of endogenous enzymes. Examples include the hot break process for tomato products (tomato soup and ketchup) to denature pectin methylesterase, which hydrolyses pectin, reducing the viscosity of the final product. Others include polyphenol oxidase, responsible for the browning of bananas, and lipoxygenase in cereals, beans, and meat, that cause rancidity.
Denaturation of Globular Proteins – Egg Proteins
How do you hard boil an egg? Conventional wisdom suggests starting with cold water near the refrigeration temperature of the eggs and heating the water, with eggs, on high until it reaches the desired temperature (most use boiling water (100oC)), after which the timer starts. Starting with cold water allows the egg to heat with the water; if eggs are submerged in boiling water, the proteins near the surface are overcooked, making the shell more difficult to remove.After that, it is pretty much preference. Egg yolk and albumen (white) contain entirely different globular proteins that begin to denature and unfold at temperatures above 60oC. This temperature allows containers of liquid egg whites to be pasteurized at 57oC for 60 min and not induce gelation.
Egg yolk pH is consistent at 6.5, while egg white pH increases from 6.5-6.7 when laid, increasing in alkalinity to pH as high as 9. The yolk membrane limits the diffusion of H+ ions allowing for the 1000-fold difference in H+ ion concentration between the yolk and white. Egg whites are ~90 % water and about 10% protein, of which ovalbumin, ovotransferrin and ovomucoid are the most abundant at 55, 12, and 11%, respectively. Before alteration, the aqueous environment causes albumen proteins to adapt a globular (3o structure) conformation, where hydrophobic amino acids are buried within the core surrounded by the hydrophilic amino acids capable of being solubilized by water. Folded proteins are stabilized by forming non-covalent (hydrogen and ionic bonding) and covalent (disulfide bridges) bonds. Electrostatic repulsion increases with protein solubility; at the isoelectric point, near pH 4.6, there is no net charge and protein coagulation occurs. Initially, egg white at pH 6.5 has a negative net charge which maintains solubility due to electrostatic repulsion between proteins, and the negative charge becomes more negative with increasing pH interacting more closely with water than other proteins.

The initial condition for the yolk is much more complex, containing lipids in addition to the water and protein and is subdivided into granules (which make it opaque) and serum, given the orange color by small molecules of Lutein and Zeaxanthin. The egg yolk plasma is mainly LDL cholesterol and livetins, while the granules are aggregates of HDL (70%), LDL (12%), and phosvitin (15%). Protein denaturation is a function of both temperature and time; while “egg timers” are set to boiling water and produce relatively reliable consistency from running yolks at 3 min in boiling water to hard-boiled at 12 min, after which they should immediately be placed in ice water to cool the temperature to prevent continued protein denaturation. Overcooking causes iron from heme and non-heme groups in the yolk to be released, which reacts with hydrogen sulfide released from the albumin to form iron sulfide at the egg white-yolk interface giving it a greenish-grey appearance. Considering the egg proteins begin to denature at > 60oC, numerous consistencies are even more tailorable using sous vide cooking techniques with exact temperature control.
The thermal energy imparted by heating weakens hydrogen bonds stabilizing the secondary and ternary protein structures while increasing polymer flexibility, causing the protein to unfold. The unfolded protein exposes hydrophobic amino acids to the aqueous phase, which is not thermodynamically favorable. Thus, the protein polymers adopt a configuration that minimizes the hydrophobic effect between segments of non-polar amino acids and water, resulting in protein-protein aggregation that effectively minimizes the newly exposed hydrophobic regions’ contact with water. Protein unfolding also exposed SH groups (Met, Cys) previously constrained in the globular structure, which can now form new covalent disulfide (first protein-S-S-protein two) bonds, making gel formation practically irreversible. Gel formation or solidification of proteins occurs when equilibrium shifts to Van der Waals attractive forces caused by newly exposed hydrophobic amino acids from the electrostatic and steric repulsive forces allowing proteins to aggregate.

Like all proteins, eggs are denatured in various ways that alter the electrostatic repulsion between proteins. Eggs covered in salt will gel in days, immersed in acid sets the protein gel in days to weeks, and coated in lye (alkali) gels in weeks to months. Each of these alters the proteins uniquely, resulting in very different gels. Salted eggs shield the electrostatic charge of the protein, allowing protein-protein interactions, thereby decreasing its solubility and allowing them to unfold and interact between multiple protein hydrophobic regions, forming a continuous, almost transparent gel with a jelly-like consistency. Pickled eggs slowly acidify until they reach a pH of ~4.6, the isoelectric point where the protein carries no net charge. Eliminating the negative electrostatic repulsion in the zwitterionic state allows the positive charge on one protein to form a non-covalent ionic bond to the negatively charged region on a second protein which then interacts with its positively charged region to a third denatured protein and so on.

Alkali or century eggs take the longest to gel, covering the eggs in alkaline salts such as ash and lye, which contain potassium bicarbonate, sodium carbonate, sodium hydroxide, and/or calcium hydroxide, increasing the pH to 9-12. At this pH, lipids and proteins begin to degrade and fragment, causing the yolk to turn green with a smooth consistency and hydrogen sulfide aroma, while the albumin turns into a dark brown translucent firm gel. Globular proteins are also sensitive to high hydrolytic pressures (>500 Mpa), causing them to denaturation by causing void volume within the globular structures to compress, altering both secondary and ternary structures and forming new non-covalent protein-protein interactions.
Denaturation of Fibrous Proteins – Collagen
Fibrous proteins are typically structural, with silk, collagen (the most abundant protein in the body), and fibrin commonly described. Skeletal muscles, or meat, are complex meshwork of collagens (more than 40 types found in connective tissues), glycoproteins (myelin sheaths), proteoglycans (filler), actin, myosin and elastin. Collagen accounts for >5% of the skeletal muscle, and when meat is cooked, it causes collagen to denature, causing the dense triple-triple (ternary (3o) structure) helices (secondary (2o) structures) to unfold into gelatin (denatured collagen) and interact with water, causing the meat to tenderize and gelatinize; however, at cook temperatures, hydrogen bonding is weak; meat rested after cooking allows water to bind more closely to the denatured collagen, which prevents fluid loss upon cutting. Collagen is also extracted from the ‘non-edible’ byproducts of the meat industry, including skin, organ meat, cartilage, and bones, which all have high concentrations.
Heat and water added during extraction are either acidified (type A) or alkalified (type B), causing collagen proteins to denature and undergo slight hydrolysis, producing gelatin soluble in hot water. Gelatin, added at low concentration, becomes soluble when heated, allowing hydrogen bonds to weaken between protein segments and water migrates between unfolded polymers. Upon cooling, the non-polar nature of the molecule tries to fold in such a way as to shield hydrophobic groups. Over short segments, the protein can form helical structures with other gelatin polymers, but the helices formed are much less ordered due to hydrogen-bound water preventing protein intra- and intermolecular hydrogen bonding.

This interaction eventually creates an entangled network that holds water by surface tension and capillary forces. Jell-O and gelatin gels are thermo-reversible because they are driven to interact by enthalpy (formation of non-covalent bonds) and not covalent bonds (egg whites and gluten). Gelatin is also very sensitive to solvent polarity, which is why only so much alcohol can be substituted when in Jell-O recipes.