Pillars of Preservation
The primary aims of food preservation are to eliminate food hazards, including chemical, physical, and biological, extend the shelf-life by limiting endogenous enzymes and spoilage microorganisms, and improve quality, which may encompass improved functionality, nutritional profile or organoleptic appeal, to ensure availability of a variety of convenience foods. Food preservation must inactivate the endogenous deteriorative enzyme causing lipid oxidation and rancidity, enzymatic browning of polyphenol oxidase, and pectin methylesterases (PMEs), which soften produce, especially tomatoes and strawberries. Additionally, it must inactivate pathogenic and spoilage microorganisms present or the environment manipulated such that they can proliferate. Once the first two are achieved, the food must be stored in such a way as to prevent contamination before use and entails food packaging, distribution and home storage (e.g., refrigeration and freezing).

Food preservation is broadly subdivided into chemical (e.g., salting (aw), smoking and curing (nitrates, nitrites, sulfates)), biological (fermentation (organic acid, CO2, ethanol)), thermal, and non-thermal technologies (e.g., high-pressure processing (HPP), electric field, irradiation, UV, cold plasma (ionized gasses)). Techniques that preserve food damage beyond repair, DNA, denature essential biological enzymes or alter the porosity of the cell membrane, all of which lead to cell death. While many aspects of food processing could be elaborated on and are of interest in the food industry, the primary focus herein will be limiting foodborne illness caused by microorganisms.
Foodborne Illness, Infections and Intoxication
Foodborne illness is any illness caused by the ingestion of food. The causative agent can be chemical (pesticides, melamine, antibiotics, cleaning and sanitizing fluids), physical (glass, metal, plastic) or biological (bacterial, parasitic, viral or a toxin produced (e.g., mycotoxins and botulinum toxin)). Viral foodborne pathogens include Hepatitis A and Norovirus, while parasitic infections are caused by Campylobacter spp (uncooked poultry), Giardia spp (water), Cryptosporidium spp (water), and Trichinella spp (more common in uncooked pork). While best practices are in place to limit microbial access to our food supply, it is under constant risk as microorganisms, including pathogens and spoilage organisms, naturally-occur in the environment we grow agricultural commodities.
Foodborne infections are caused by food-containing bacteria colonizing the intestinal tract. As a result, epithelial cells are damaged and unable to take up solutes leading to an osmotic gradient that causes water to move from tissues back to the intestinal tract (diarrhea). Gram-negative (Salmonella spp, e. coli Yersinia, Shigella) bacteria induce a pyrogenic effect (fever), causing a fever due to lipopolysaccharide and disease onset ranges between 12hr to 2 days and is characterized by watery diarrhea. Gram-positive bacteria Listeria monocytogenes, a psychrotrophic bacteria (cold tolerant), is notoriously associated with deli meats, causes Listeriosis when septic and through pregnancy, results in fetal loss (~20*) and newborn death (~3%). Staphylococcus aureus is another example of a Gram-positive bacteria causing foodborne illness, and the emergence of multi-drug resistant strains such as MRSA (Methicillin-Resistant Staphylococcus aureus) is of significant concern, but the routes of infections are not primarily food.
Foodborne intoxication occurs when bacteria produce toxins in the food or during colonization (intoxication), causing very fast onsets times (12-16 hrs)> Intoxications include Clostridium botulinum, Clostridium perfringens, and Bacillus cereus, all of which are Gram-Positive spore-forming rods. In anaerobic containers at pH > 4.6, sporulation of c. botulinum releases the botulinum toxin into the food and small amounts of ingested toxin, also dubbed the miracle poison, blocks acetylcholine release between neural synapses, resulting early on in blurred vision and progressing to difficulty breathing and eventually cardiac arrest when left untreated. In the case of b. cereus, intoxication results from ingesting the emetic toxin, while intoxication results from diarrhoeal toxin released during a toxin-mediated infection. Verotoxin-producing Esercheri coli (VTEC) is another toxin producer but is gram-negative.
Intrinsic and Extrinsic Factors that Affect or Control Microbial Growth
Bacteria may remain dominant until a hospitable environment is present, and after a short lag, bacterial growth is exponential (logarithmic), typically doubling every 20 min under ideal conditions. Foods are made commercially sterile by understanding how to manipulate the intrinsic parameters of food and its extrinsic storage conditions. Intrinsic parameters are inherent to the food and include biological barriers that are physical such as peels, skin and shells; pH and small organic acids (acetic, propanoic and lactic), which are bacteriostatic (prevent microbial growth) at ~1% and bactericidal at 2% (kills microbial cells); water activity (aw) or available water to support microbial growth, and finally antimicrobial constituents. Extrinsic parameters include the temperature of storage, the presence of oxygen, and the relative humidity.
Effective barriers to microorganisms include eggshells, shells on nuts and seeds, citrus peel, fruit skin, and rinds of melon and the skin of livestock. It is safe to assume that those same surfaces are contaminated with undesirable microorganisms, making their removal of utmost importance. For example, the evisceration of cows and pigs removes the entire gastrointestinal tract (from the esophagus to the anus) intact, and after the skin is removed, only the surface of the meat is exposed to microbes. A stake from a cow can safely be eaten after searing the surface, leaving the internal temperature medium-to-rare, while comminuted (reduced to small particles) meat and pork (risk of parasitic oocytes through the meat) must be cooked well-done to ensure the food is safe to consume.
Organic Acids, and pH as Intrinsic Parameters
Food science has a very clear delineation concerning pH and microbial risk – that pH is 4.6. High-acid foods (pH < 4.6) limit the microorganisms that can survive during thermal processing. Under anaerobic conditions (packaged food with hermetic seals (e.g., cans or tetra pack containers)), Clostridium botulinum endospores germinate back into vegetative cells, producing the botulinum toxin only when the pH of the food pH > 4.6.

pH is extremely important when canning foods, including preserves like jams and jellies, fruit and vegetables, meat products, and soups. To preserve high-acid foods, hot filling (100oC) followed by sealing and inverting the container is sufficient to preserve the acidic food. To safely preserve low-acid foods, which are many soups and meats, a much more intense thermal process must be applied to ensure c. botulinum spores do not germinate back to vegetative cells.
While small organic acids (lactic, acetic, propionic, etc.) alter pH, their role in preserving foods arises due to their ability to transport through cell membranes to the microorganism cytoplasm. During fermentation, desirable, often probiotic microorganisms consume glucose and, as a product, form different organic acids. Organic acids are bacteriostatic, inhibiting microbial growth at ~1%, and bactericidal, killing bacteria above 2%. While microorganisms are very effective at preventing ionized small molecules from crossing the cell membrane, protonated small organic acids can pass the cell wall and enter the cytoplasm.
Organic acids, either added to food, naturally found in food, or produced during fermentation, function when the pH of the food is near or below the pKa of the acid. In the case of yogurt, during fermentation, the pH of milk decreases to between 4.4 and 4.8 as glucose is converted to lactic acid (pKa = 3.8), resulting in approximately 40% of the lactic acid being in the protonated form and 60% being deprotonated. As the lactic acid passes into the microbial cell, the cytoplasm pH is ~6.0, causing lactic acid to deprotonate and acidify the cytoplasm. Using energy (ATP), the microorganism can compensate by pumping excess hydrogen ions out of the cell via efflux pumps.

The microorganisms survive at low concentrations of ~1% but cannot replicate because all available metabolic energy is used to maintain the cytoplasm pH (bacteriostatic). When the concentration of organic acid is higher, ~2%, it accumulates in the cell faster than the efflux pumps can remove, and the cytoplasm acidifies denaturation essential enzyme resulting in cell death (bactericidal).
Water activity (aw) as an Intrinsic Parameter
Water activity is the ratio of the fugacity (f) of water in food compared (fo) to the fugacity of pure water, and fugacity represents the tendency of water to escape from the system and closely relates to vapor pressure; hence water activity may be rewritten as: aw=f/fo ~ p/p*i
where is the vapor pressure established above the food and pi* is the vapor pressure of pure water at the same temperature. Adding solutes and macromolecules to water decreases the entropy of the solution relative to pure water, resulting in a decreased vapor pressure, a colligative property. Materials have an aw between 1 and 0 (100% and 0% relative humidity) for pure and no water systems, respectively. Water activity, aw, correlates with microbial growth, deleterious reactions, oxidations, and vitamin and color degradation.
Water activity affects food quality and stability, including microbial spoilage and enzymatic and non-enzymatic deteriorative reactions. The aw of food is reduced by adding humectants (bind water) or by removing water during drying. Water in foods at, or below, 15% is bound in the BET monolayer and corresponds to low aw, as the water is closely associated with monolayers and multilayers and does not participate in hydrating microbes nor partaking in the mobility of enzymes. Intermediate moisture foods (IMF), with 20 to 40% moisture, exceed monolayer and multilayer water; water is somewhat confined as capillary water. IMFs are highly susceptible to enzymatic reactions and, to a lesser extent, microbial spoilage. High moisture foods, > 40% moisture, undergo rapid spoilage if no alternative processes have been applied to limit deleterious reactions from occurring. aw predicts the occurrence of different types of food spoilage; for example, at an aw less than 0.65, no microbial spoilage occurs, which exponentially declines with decreasing aw. Gram-negative bacteria are adapted to low osmolarity and found in highly dilute foods, unable to grow in foods with a water activity less than 0.97; while gram-positive bacteria can survive and proliferate at water activities as low as 0.9. The outer lipopolysaccharide for Gram-negative bacteria restricts water and nutrients from transporting into the cells making their water requirement greater than Gram-positive bacteria. Yeast and molds survive in lower moisture foods than bacteria and tolerate lower water activity 0.7. Not all correlations between water activity and spoilage rates are as simple as that observed for microbial growth. For example, lipid oxidation occurs at the slowest rate when the aw is near 0.2 and is attributed to small amounts of water required to bind hydroperoxides; and more water added to the system aids in solubilizing oxygen into the food matrix, increasing the rate of oxidation to a maximum at an aw of 0.75, after which the high water activities dilute substrates effectively slowing oxidation. The Maillard reaction exhibits maximum activity at an aw of 0.75 due to feedback inhibition when water is an end product.
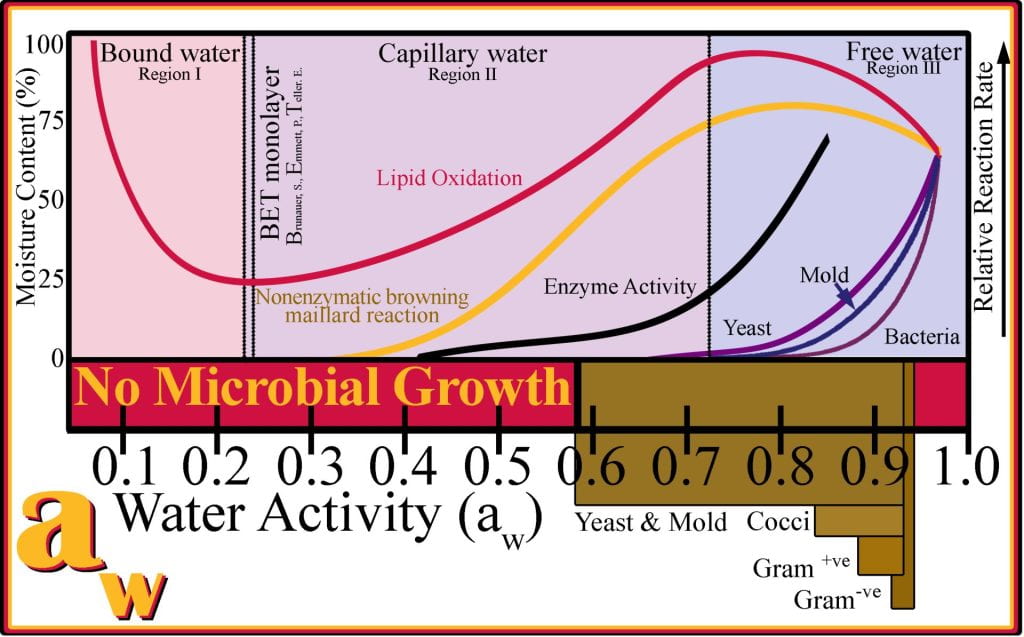
Storage Temperature as an Extrinsic Parameter
Many fresh foods require reduced storage temperature to limit the growth of microorganisms. One of the greatest advancements affecting our food supply was the first home refrigerator in 1913, allowing food to be preserved longer than at room temperature. Since the human body is 37oC, most pathogens that cause illness are mesophilic; thus, reducing the temperature to below 5oC extends the shelf life as mesophilic growth slows, and when frozen (-10oC home freezer), they become dormant. Psychrophilic, including pseudomonas, micrococcus, and mycobacterium, can still replicate and cause spoilage and illness. Also important to consider is that refrigerating foods can induce undesirable changes at low temperatures, as seen in the chill injury of bananas and cold staling of bread. Once foods have been cooked, leftovers must be cooled through the danger zone 10-55oC, where rapid cell multiplication occurs, to below 5oC for it to remain safe to consume. Leftovers that have remained in the danger zone should only be consumed after cooking it to an internal temperature of 75oC or above to kill any bacteria present.

Headspace Gases (aerobic or anaerobic) as an Extrinsic Parameter
The gas surrounding the food, if in a package or can, alters the microorganisms that can survive. Early manipulation of headspace gas involved canning and creating a hermetic seal to ensure oxygen cannot enter, inhibiting the growth of aerobic bacteria (requiring oxygen for respiration) while facilitating the growth of anaerobic bacteria. Active food packaging plays an integral role in maintaining headspace and either slowly releases or absorbs compounds to maintain a specific set of environmental conditions beyond simple aerobic or anaerobic environments. In addition to controlling microbial growth, ethylene gas initiates the final ripening of fruit, or the ethylene absorbent package can absorb the naturally released ethylene extending the self-life.
Food Packaging
In addition to maintaining the headspace gas as an extrinsic parameter, packing also serves as a barrier to microbial contamination post-processing, moisture into or out of the food, and oxygen and UV light, which are essential in slowing the oxidation of unsaturated lipids. In addition to maintaining product quality, it relays nutritional information, ingredient list, and allergens; in some instances, it allows visual evaluation of the food. The need and ability to preserve food occurred before there was an understanding of what spoiled food. During the Franco-Russian wars, Napoleon Bonaparte offered a reward to anyone who could preserve food to reach the frontlines allowing troops to advance during seasons when food was unavailable to plunder. Nicolas Appert developed Appertization by preserving foods in continuously sealed glass bottles, wrapped in cloth and heated in a pressurized vesicle (early retort) to prevent spoilage. At this point, spoilage organisms were unbeknownst to humans and Aristotle’s scientific theory of spontaneous generation that living creatures could arise from nonliving matter and was commonplace until later works of Louis Pasteur.

However, glass bottle presents numerous challenges, with the most obvious being fragile, and when it breaks, there is no simple way to remove glass shards. Not without its shortcomings, the glass bottle, for the most part, especially for foods, moved to the three-piece tin can, which would be soldered using a mixture of tin-lead solder. Once toxicity became associated with the lead used in soldering tins, the industry had to find a way to create a can without the need for soldering. Hermetically (airtight) sealed cans today have been used since before the 1900s and have a two-piece construction. The bottom and sides of the can are stamped in one piece from sheet metal, while the lid was a separate piece. The can’s top and lid are flanged downward, and the can is sealed in two steps. The first step rolls the lid flange over the cans, and the second step continues the seal until the can body is in tight contact with the lid twice. If formed correctly, it is impervious to the elements; however, any dent or damage to the cans’ seal means it contains potentially harmful microorganisms and should be discarded.
With the advent of out-of-package processing (aseptic processing) also came laminated packaging or multilayered containers (Tetra Pak) as they do not have to tolerate the same intense thermal process a can must. Tetra Paks can be filled continuously during stealing; when one package fills, it is closed with a double-sealed using thermal contacts, one seal is the top of the full package, and the other is the bottom of the next container, which is filled and sealed, forming the top and the bottom of the next container.
Layers of material held with adhesive typically contain a food-contact layer which can be inert or active. Inert packaging should not allow volatile aromas, flavors, or acids to be absorbed from the food, nor should it leach components into foods. Active packing releases more often than absorbs intended compounds, often targeting microbial growth. The most common food contact layer is a double layer of polyethylene, as it is a good moisture barrier. A layer of Aluminum acts as a good oxygen barrier, and then the paperboard blocks UV light and holds the ink layer. The last layer, usually polyethylene, protects the paperboard from high relative humidity (water in the air).

Food Packaging-Milk Bags?

Why milk is sold in bags may seem odd, but it was introduced because it was a low-cost solution when legislation was passed, switching from the imperial to the metric system on April 1, 1975. This meant reusable, thick imperial glass bottles used before 1975 would have to be eliminated from circulation and forming equipment in metric sizes would need to be sourced, a cost the dairy industry could not afford. Dupoint had been using polyethylene bags throughout Europe, and the durability of the packaging compared to glass bottles appealed to the dairy industry. Consumers still benefit from being able to access quality visually, and it does not allow an anaerobic environment to form as it is a poor oxygen barrier. This element is key since milk is pasteurized and not commercially sterilized; therefore, pathogen mesophilic bacteria are the target organisms, which is also why milk in bags still needs refrigeration.
Hurdle Technology and Eliminating Foodborne Illness
Today’s foods combine numerous sublethal intrinsic and extrinsic modifications to the food, each of which impedes microbial growth but does not produce a commercially sterile food. Hurdle technologies combine methods to eliminate or control the growth of pathogens, extending its shelf life while ensuring the food safe for consumption. Intrinsic parameters of the food, such as pH, water activity (aw), and the presence of organic acids, salts, nitrates, nitrites, phosphates, or sulfates, all impede some bacteria from surviving in food. Extrinsic parameters applied to the food can include electromagnetic (ultraviolet, infrared and microwave processing), irradiation (ionizing), thermal processing, refrigeration and freezing, and packaging, including the modified atmosphere composition (N2, O3, CO2) and redox potential (aerobic vs anaerobic). Combining sequential hurdles applied to foods ensure that microbial growth is not possible under those conditions.

Contents