Refining of Sucrose From Sugarcane and Sugar Beets
Simple sugars, including mono and disaccharides, are found in many whole foods; however, there are limited sources to obtain purified ingredients through extraction and refining. The major sources of commercial refined sugars include sucrose from sugarcane and sugar beet, lactose valorized from dairy waste streams, and glucose, fructose and maltose refined from corn and cereals. Syrups such as maple, agave and honey contain more water than crystalline sugar and a greater diversity of sugars. Honey contains glucose and fructose, while maple syrup contains sucrose, glucose, fructose and complexes thereof, manganese, zinc, magnesium, calcium and potassium, vitamins and polyphenols.
The vast majority of sucrose is supplied by sugarcane plantations producing approximately two billion tons annually, while sugar beet account for ¼ billion tons and is processed using similar methods. Raw products are crushed, causing cellular decompartmentalization to facilitate separating fluids (70% total mass) from the remaining bagasse (~30% total mass). Solids in the sap following crushing are filtered out, and the remaining sap is boiled to increase the concentration of sugars, and some impurities are skimmed off the surface as they form a froth. The bagasse is often used as fuel to boil the sap. Granular sugar is added after boiling and cooling the solution, seeding crystal growth and allowing the supersaturated sugars to precipitate onto the added sucrose crystals alongside many impurities. Cane sugar has many names depending on location rapadura (Brazil), jaggary (India), panela (Latin America), and kokuto (Japan) and also non-centrifuged cane sugar, as this is the first major delineation between other processed sugars.
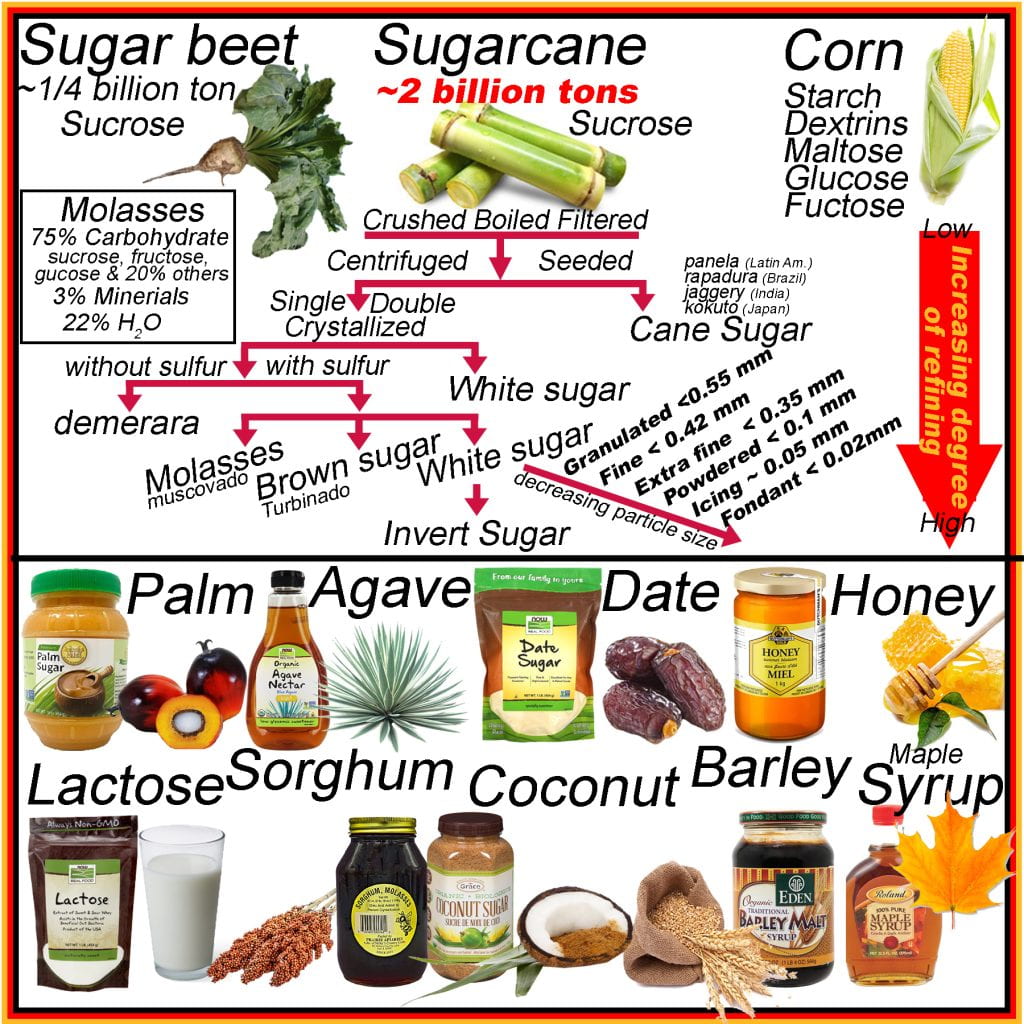
Instead of seeding to produce cane sugar, the cooled solution is refined by centrifugation with or without adding organic sulfur to prevent off flavors from forming. Without adding organic sulfur, demerara is produced, which has a bitter flavor. Ensuing centrifugation, crystallization and a second stage of centrifugation, pure crystalline sucrose (white sugar) is obtained by separating the molasses from the sucrose in cane sugar. While molasses, or muscovado, is primarily sugar, it also contains minerals, water and other compounds, making it appear dark brown to black. Brown sugar or turbinado contains both molasses and sucrose.
The granular white sugar (~0.5 mm) is pulverized to different extents depending on the application. White sugar is commonly available, in order of decreasing particle size, as fine (<0.42 mm), extra or ultra-fine (< 0.35 mm), powdered (< 0.1 mm), icing (0.05 mm) and fondant (0.02 mm). Sucrose or white sugar may be treated with invertase to obtain glucose and fructose (or invert sugar). Invert sugars are termed so due to the optical rotation of light for sucrose compared to glucose plus fructose is the opposite.
Refining Starch
Carbohydrates from corn, cereal and potato are high in the polysaccharide starch and undergo different processing steps to acquire the starch granules. For corn, once cleaned and de-husked, the kernels are steeped at ~ 50oC in slightly acidic water, allowing water to absorb, increasing the moisture content from 15 to 45% and doubling the kernel volume. The acidic water weakens gluten bonds causing the starch to release, after which the corn is coarsely ground, breaking away the germ layer. Cyclone separators remove the low-density (~ 85% of the oil) germ, which is washed and screened to ensure all starch is removed, then the germ layer is further refined into corn oil. The remaining corn and water slurry is impact-milled, a much more vigorous grinding than previous, to release the starch and gluten from the fiber in the kernel, which is then screened and washed out, leaving a starch-gluten suspension or mill starch. The starch-gluten suspension undergoes centrifugation separating the lower-density gluten protein from starch. At this stage, the starch slurry contains < 2% protein, which is repeatedly washed and separated using centrifugal separation, leaving >99% starch after drying, and the product produced is unmodified corn starch.

Carbohydrates from corn, cereal and potato are high in the polysaccharide starch and undergo different processing steps to acquire the starch granules. For corn, once cleaned and de-husked, the kernels are steeped at ~ 50oC in slightly acidic water, allowing water to absorb, increasing the moisture content from 15 to 45% and doubling the kernel volume. The acidic water weakens gluten bonds causing the starch to release, after which the corn is coarsely ground, breaking away the germ layer. Cyclone separators remove the low-density (~ 85% of the oil) germ, which is washed and screened to ensure all starch is removed, then the germ layer is further refined into corn oil. The remaining corn and water slurry is impact-milled, a much more vigorous grinding than previous, to release the starch and gluten from the fiber in the kernel, which is then screened and washed out, leaving a starch-gluten suspension or mill starch. The starch-gluten suspension undergoes centrifugation separating the lower-density gluten protein from starch. At this stage, the starch slurry contains < 2% protein, which is repeatedly washed and separated using centrifugal separation, leaving >99% starch after drying, and the product produced is unmodified corn starch.
Cleaned potatoes or tubers are rasped (shredded) with sulfur dioxide to prevent enzymatic browning, and the mash is sieved to remove fiber. In the mash fluid, starch granules are liberated into the fluid that also contains phenols and proteins, which in the presence of oxygen, undergo undesirable non-enzymatic browning reactions prevented by sulfur dioxide addition. The mash is repeatedly washed in aqueous sulfuric acid, and the starch fraction (starch milk (60% water)) is separated using centrifugation (cyclones). The starch milk is dried to a moisture content below 20% producing starch granules that are 50 to 100 nm and trace calcium, protein, potassium and iron.
Wheat milling today varies from coarse-ground whole flour produced by a single-pass rotating stone mill to much more advanced refining that purifies only the starch granules containing amylose and amylopectin. Advanced milling undergoes successive gap reduction between rollers; the first roller breaks the bran layer from the endosperm from the germ layers. The powder is sieved, removing fiber before the next size reduction by passing it through the successive rollers with a narrower gap. The subsequent powder is sieved with a smaller sieve pore size that removes the germ layer, and flour (90% starch & 10% protein) remains.

Edible Oil Refining

Except for animal products and olives, plant and single-cell oils are obtained by refining after crushing, which purifies the TAGs from the crude mixture of lipids and aliphatic molecules. The crude oil is mixed with hot water to first degum the oil. Degumming removes the polysaccharide gums and other impurities, such as phospholipids and proteins, all found in cell wall fragments, using hot water or dilute acid. When mixed with hot water, the active surface and more polar molecules found in crude oil are solubilized into the aqueous phase, which is then centrifugated, separating the gum from the now-degummed oil. Next, neutralization removes free fatty acids from the degummed oil by adding caustic soda (NaOH). The alkalinity ensures the fatty acids are deprotonated, allowing them to form fatty acid soaps and be removed with water via washing.
Neutralized oil is now free of gums and fatty acids. Neutralization improves the flavor and stability of the oil. The neutralized oil undergoes bleaching, which is the adsorptive purification of oil by passing it through a diatomaceous earth bed under a vacuum, allowing for deodorization to remove volatile compounds (aroma). Diatomaceous earth is a naturally occurring, soft, sedimentary rock high in silica (used in chromatography) comprised of the fossilized remains of microalgae (diatoms). The high silica material easily crumbles into a fine powder with high porosity, thus effectively reducing the contents of chlorophyll, residual soap and gums, trace metals, and oxidation products, which improves flavor and color and increases shelf-stability. Bleaching efficacy relies on the processing variables temperature, humidity, contact time, gauge strength of the vacuum, oil quality and moisture, and the characteristics of the adsorbent bed. During this stage, fatty acids soaps are completely removed, iron and phosphorus are reduced to < 0.2 ppm, chlorophyll to < 0.05 ppm, and the peroxide value is <0.5 mEq/kg ( which is the milliequivalents (meq) of active oxygen per kilogram of oil). Winterized oils undergo an additional step that cools the oil to crystallize high melting point TAGs so it can be stored at refrigerated temperatures without turning cloudy from forming small crystals, which is important for manufacturing salad dressing. Finished oils include store-bought and industrially- used corn, canola, sunflower, olive and vegetable oils. It is also important to note that extra virgin and virgin olive oils are the first and subsequent crushes of olives, and it is not purified further to separate the pigments, which is why olive oil has an as different color than other oils. After olives have been pressed, the remaining olive material will be solvent extracted to produce olive oil (without the designation ‘virgin’ or ‘extra-virgin’).
Milk Fat Separation-Centrifugation
Milk fat composition varies based on breed, genetic variation within the breed, animal health, environment, management practices, and diet; however, the prominence of the Holstein breed leads to a fairly consistent composition of 3.6 % fat, 3.2 % protein, and 4.7 % lactose. Milk has the highest fat content after birth in the colostrum, followed by a steady decline over the first two months of lactation, after which the fat content slowly increases through the rest of lactation. Through the first half of lactation, the short- and intermediate-chain fatty acids increase in concentration while long-chain fatty acids decrease, which remains constant through the latter half of lactation. In Canada, milk pricing is based not on the fluid weight but on a lipid and protein component basis. Therefore, all milk products in Canada undergo standardization, separating the lipid component from skim milk followed by blending the skim milk and cream to provide the various exact milk fat contents of 1, 2 and 4.25% whole milk and 18 and 35% creams. Milk fat is separated by centrifugation, where the centrifugal force is applied to the milk by spinning it rapidly in a centrifuge, causing the milk fat particles to separate from the more dense aqueous phase.
A centrifuge has as many as 120 perforated, 45 to 60-degree-angle discs with 0.4 to 2.0 mm separation channels. Prior to, or concurrently with, separating the fat, milk is clarified using a lower angular velocity or desludging mechanism to remove solid impurities, including cells and bacteria, from the milk. Separating fat from milk relies on creaming, characterized by Stoke’s law, due to the density difference between the lipid and water phases.

Creaming is accelerated by increasing the gravitational force (F=mω2r) via centrifugation from 9.8 m/s2 to 1000 to 5000 x the force of gravity (g), or between 10,000 to 50,000 m/s². The centrifugal force is a function of the centrifuge’s radius (r) and angular velocity (ω). Milk is introduced at the inner edge of the disc stack with vertically aligned holes; the less dense fat globules move inwards through the separation channels toward the axis of rotation. The process efficiently removes fat from skim milk (0.1% fat); however, skim milk is needed to carry fat globules, and the cream content reaches a maximum of 35-40% milk fat. Additional processing is required to increase the milk fat concentration beyond 40%.
Milk Fat Separation-Churning
The cream is aged at 18 oC before churning to ensure fat crystals are present in the lipid droplets, while at the same time, they are not too solid to prevent coalescence. The milk fat concentration of cream is further increased by churning to form butter which is ~82% lipid, ~16% water, and the remaining 2% is protein, carbohydrate, and minerals; the typical fat composition is 65% saturated, 27% monounsaturated, 4% polyunsaturated, and 4% trans-unsaturated fatty acids. Butter is one of the most complex lipid sources, with over 100 fatty acids synthesized by the rumen microflora and incorporated into the milk fat. Butter churning uses an impeller designed to agitate cream, a high-shear process incorporating air. Air bubbles form and implode during this process, creating smaller fat droplets; as the droplet size decreases, insufficient milk fat globular membrane is present to cover the entire fat drop and coalescence of fat droplets occurs. After churning, the material enters a twin-screw impeller which adds further shear to increase fat coalescence; throughout the separation, buttermilk is removed from the butter and, done under vacuum, to remove air from the final product while preventing oxidation. After separation and the buttermilk is drained, working establishes a continuous fat phase containing a finely dispersed water phase (butter) by further shearing the material under a vacuum.
While there are more sophisticated methods to isolate and purify milk fat, most of the fluid milk is centrifuged, and standardized to kinds of milk and creams, while further fat isolation is done by churning cream into butter. Ghee further concentrates lipids from butter by heating butter to 105-115oC; as the mixture simmers, the remaining proteins float to the surface and are removed. Heat is applied until the mixture is clear with no remaining solid on the surface, and the clarified oil is decanted off the more dense solids that have precipitated to the bottom. The clarified butter is then heated to 130 oC, which triggers the Maillard reaction between lactose and residual proteins creating the light brown pigments while eliminating the carbohydrate and protein, during which the fat content to 98.9 % fat, with trace water and non-fat solids (products of the Maillard reaction).

Valorization and Molecular Refining
Unused or waste products account for 1.3 billion tones (3.3 billion tones of CO2) of agri-food which can be diverted from landfills or incineration by valorizing them to reduce the environmental footprint and increase the value of unused parts of single-cell organisms (e.g., bacteria, yeast, mold, fungi, algae), plants, and animals. Valorization produces end-use products often added to the food supply or products from other industries with functional or nutritional benefits. Valorization converts food waste to food, feed, fertilizer, or fuel and molecules extracted are added to essential oils, cosmetics and personal care products, packaging materials and substrates for fermentation. A simple example of valorization is composting spent coffee grounds, of which only 30% of the beans mass solubilizes during brewing, leaving 70% of the mass, which may be added to soil as compost which slowly releases nitrogen and is known as the ‘ground to ground’ initiative. Alternatively, individual components, such as lipids, proteins, carbohydrates, bioactives and nutrients, can each be isolated by combining various sequential size reduction, extraction, isolation and modification methods converting the waste into biofertilizers, bioplastics, biofuels, chemicals, and nutraceuticals.